Researcher Spotlight
Seeing the invisible – Capturing single photons in the infrared - MIT-Harvard Center for Excitonics
Raoul Correa, a fourth year graduate student, is a perfect colleague. Most of the work within the Center for Excitonics occurs among scientists that are in many ways joined at the hip, and in many ways his research epitomizes this. A physical chemist in Moungi Bawendi’s group at MIT, Raoul’s work is focused on exploring the potential of nanomaterials as absorbers of light from various parts of the solar spectrum. An understanding of basic physical processes at the nano-scale is critical for improvement of real-world devices, such as more efficient light bulbs, and less expensive solar cells. To tackle this problem, Raoul and his team utilize special techniques to probe novel nanomaterials in a very direct manner, studying fluorescent molecules one at a time. The results are important because they tell us how electrons (and their counter-part, holes) interact inside potential solar materials, and guides rational device fabrication being done by other scientists at MIT and beyond.
All the research groups within the Center for Excitonics are concerned with the exciton’s lifecycle –excitons are charged carriers – from the point of generation, transport, and eventual conversion into electricity. To study and optimize this process, a number of new technologies are explored and invented at the same time. This includes the construction of new apparatuses, something the Center can do easily given its combination of chemists, electrical engineers and physicists. A good example of the marriage of technology and fundamental research is Raoul’s experiment – a novel technique that allows researchers to characterize the exciton lifecycle in the infrared, a relatively unexplored area of the spectrum.
The subject of examination is the colloidal quantum dot, a chemically synthesized material with large absorption and readily tunable optical properties. A distinct advantage is that QDs are easily manipulated: scientists can simply change the size of the particle and achieve light absorption and fluorescence right across the visible and infrared portions of the spectrum. Scientists call this “size-band gap tunability”, giving QDs a unique edge and holding some promise that QDs could be used to absorb nearly all of the solar radiation spectrum.
It turns out that studying the fluorescence from QDs reveals a great deal of information about excitons, even more so if one can look at these nanomaterials one at a time. To do this, Raoul established for himself a particular challenge: he sought to view, one-at-a-time, infrared photons that originate from infrared emitting QDs, a potential solar-active material. To begin to understand Raoul’s research it’s important to know that for some time, scientists have been working on extending traditional infrared detection technologies based on silicon, and also InGaAs, an expensive semiconductor alloy, to detect infrared light at the single photon level. Their efforts to do this have been frustrated because they were restricted to a small subset of very bright samples, and could not use the same experimental techniques on weak emitters that are more pervasive (like molecules, or colloidal QDs). MIT’s novel contribution, in collaboration with Lincoln Labs and Karl Berggren’s Quantum Nanostructures Nanofabrication group, is to use a radically different approach that circumvents the sensitivity problem.
Armed with a sensitive detector in hand, the team could get to work. When light hits the QDs, they fluoresce or glow and this emission is collected and measured by a superconducting nanowire single photon detector, recording the precise time that a photon was detected. In the first “proof of concept” experiment, Raoul and his collaborators successfully measured single infrared photons from single QDs, an exciting step forward. The team is cautiously optimistic about these results and currently is working hard to improve the efficiency of this signal, so the technique can be used for more sophisticated experiments. Characterizing the properties of individual QDs is highly desirable, as it reveals heterogeneous behavior often obscured when studying a large collection of QDs all at once, since under those conditions it would be impossible to document the differences between varying particles.
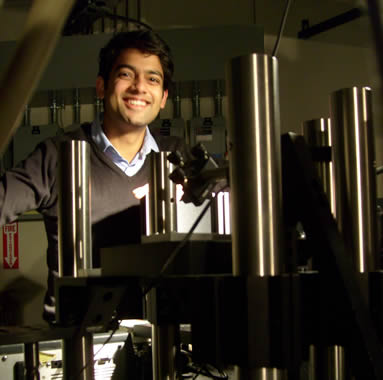
Raoul Correa standing next to a fluorescence microscope in the Bawendi lab.
There is a logical division of labor between research groups at the Center for Excitonics, given their mutual interests in the successful use of the techniques mentioned earlier. Raoul works with Prof. Berggren’s device group and a team at MIT’s Lincoln Laboratories in a symbiotic way: his observations help them to continually think of new ways to improve the detector that measures single photons, and in conversations with them, Raoul and his group are forced to think about how better materials can be synthesized to generate highly photostable materials and improve the collection of light from single infrared-emitting systems. In Moungi Bawendi’s group, he finds guidance and support for his work with lead sulfide QDs from those who are expert in the use of synthetic materials. All these interactions are facilitated by the co-location of the investigators together in the Center.
So as Raoul pushes forward with his plan to examine single QDs, Karl Berggren’s group continually refines their design and implementation of the infrared detector. The payoff is potentially huge: success in the creation of a sensitive apparatus for optical measurements in the infrared is a precursor to continuing successful work in other Center research groups, like Marc Baldo’s group studying multiexciton dynamics, to optimize absorption of energy using specific materials and reduce thermal emissions. Many investigators within the Center for Excitonics are looking for ways to directly observe the excitonic activity in their materials (see article about work by Priya Jadhav). So having the infrared detector as part of the Center opens this door to everyone.
So, how did Raoul prepare for his work as a physical chemist? As an undergraduate student at the University of California – Berkeley, he worked in the laboratory of Stephen Leone, a pioneer of ultrafast spectroscopy, where he studied the dynamics of electrons in atoms with high powered lasers and big vacuum chambers. He was amazed to learn that research in chemistry could be like this. Raoul says, “I thought it was just mixing chemicals and wearing a white lab coat! As a kid I was a huge fan of Lego, and this seemed like a natural extension of that, only now, people called it science. The building of large apparatuses was critical to me considering a scientific career in physical chemistry, but so was the culture of teamwork.” Raoul continues, “We’ve been tasked by society to produce results – real results that matter and inform. Investment in fundamental research and the training of young scientists is a smart bet for society to make – there are countless examples that prove this point. My colleagues and I recognize this responsibility, and we are trying to do our very best to make good on that investment.”